Even though scientists have been studying genetics since the mid-19th century, they continue to make new discoveries about genes and how they impact our health on a regular basis. NIGMS researchers study how genes are expressed and regulated, how gene variants with different “spellings” of their genetic code affect health, and much more. Get the drop on DNA and the gist of genes with these fast facts:
Continue reading “Genetics by the Numbers”Science Snippet: Breaking Down Biodegradability
Have you ever noticed plastic utensils or paper plates labeled as “biodegradable” and wondered what that meant? Materials are biodegradable when microorganisms such as bacteria can break them down into their building blocks.
Continue reading “Science Snippet: Breaking Down Biodegradability”Martin Burke: Replacing Lost Proteins to Treat Disease
As a medical student, Martin Burke, M.D., Ph.D., helped care for a young college student with cystic fibrosis (CF), an inherited disease that affects the body’s ability to make sweat and mucus. Dr. Burke had just studied CF in class, so he relayed what he had learned to her. He had a lot of information to give—doctors and researchers know the exact amino acid changes in an ion channel protein called cystic fibrosis transmembrane conductance regulator (CFTR) that cause CF.
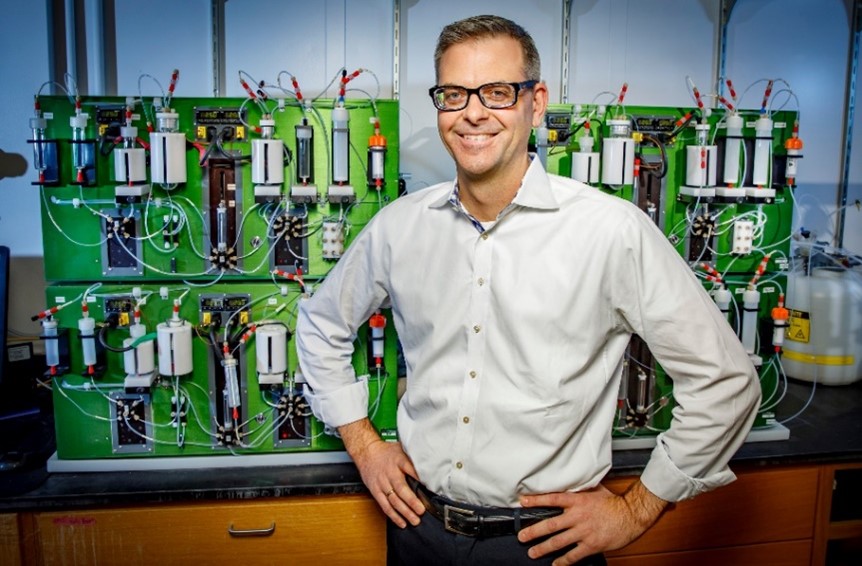
“At one point in the conversation, she stopped me and said, ‘It sounds like you know exactly what’s wrong with me, so why can’t you fix it?’” Dr. Burke, now the May and Ving Lee Professor for Chemical Innovation at University of Illinois Urbana-Champaign (UIUC), never forgot this question. In fact, it’s inspired his career-long search for new ways to develop therapies for diseases without effective treatment options.
Continue reading “Martin Burke: Replacing Lost Proteins to Treat Disease”What Is Genetics?
Genetics is the study of genes and heredity—how traits are passed from parents to children through DNA. A gene is a segment of DNA that contains instructions for building one or more molecules that help the body work. Researchers estimate that humans have about 20,000 genes, which account for about 1 percent of our DNA. The remainder of the DNA plays a role in regulating genes, and scientists are researching other potential functions.
Continue reading “What Is Genetics?”In Other Words: What Being Unionized Means for Molecules
Did you know that molecules can be unionized? But it doesn’t mean they form a labor union. In chemistry, unionized (pronounced “un-ionized”) is the opposite of ionized, which means “electrically charged.”
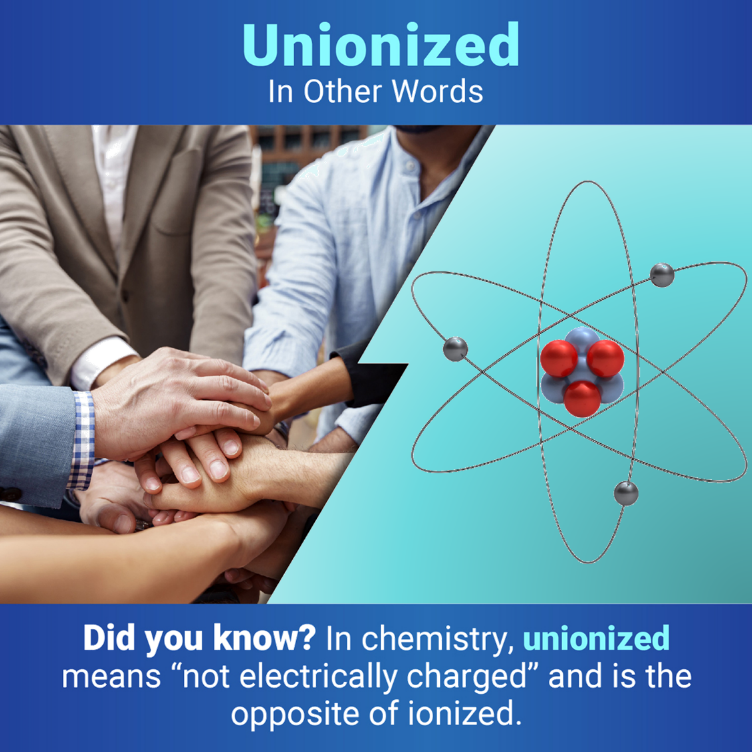
Investigating the Primary Cilium: Q&A With Xuecai Ge
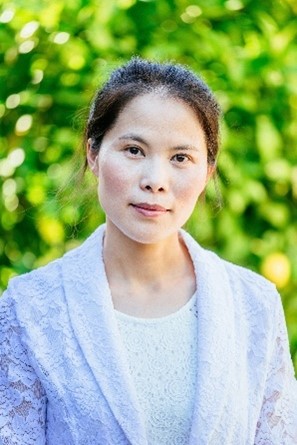
The brain is a large and complex organ, but some very small structures guide its development. Xuecai Ge, Ph.D., an associate professor of molecular and cell biology at the University of California, Merced (UC Merced), has devoted her career to understanding one of these structures called the primary cilium. In an interview, Dr. Ge shared how her childhood experience inspired her to study science and what makes the primary cilium fascinating.
Q: How did you first become interested in science?
A: When I was a little kid, my mom was a primary care doctor, and I saw her treat patients in our community. I noticed that no matter who got a particular illness, she could use the same medicine to treat them. My little mind was amazed that the same medicine could work for so many different people! I think this early experience planted the original seed of my interest in life science.
Continue reading “Investigating the Primary Cilium: Q&A With Xuecai Ge”What Is the Microbiome?
Have you ever noticed a skin care product advertised as “microbiome friendly” and wondered what that meant? The microbiome is the collection of all the microbes—including bacteria, viruses, and fungi—that live in a specific environment, such as on the skin or in the digestive tract.
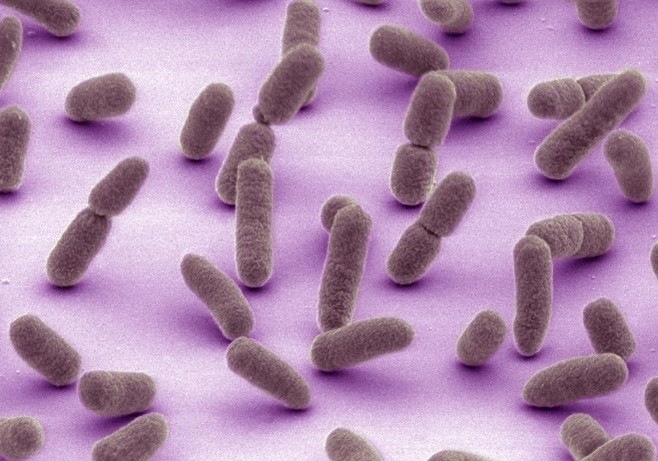
Credit: Mark Ellisman and Thomas Deerinck, National Center for Microscopy and Imaging Research, University of California, San Diego.
It’s a common misconception that all microbes are harmful—in truth, much of the human microbiome is made up of microbes that form beneficial symbiotic relationships with us. Microbiome-friendly skin care products don’t have antimicrobial properties that could harm the beneficial bacteria that live on our skin.
Your Microbiome and You
Continue reading “What Is the Microbiome?”Science Snippet: Examining Enzymes
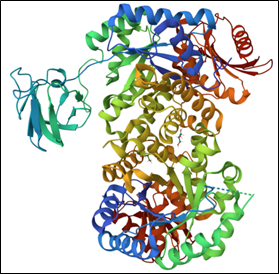
Every day, our cells must produce all the various molecules they need to stay alive. But the chemical reactions to create these molecules can’t occur without help—which is where enzymes come in. Enzymes are biological catalysts, meaning they speed up the rate of specific chemical reactions by reducing the amount of energy needed for the reaction to occur. Most enzymes are proteins, but some RNA molecules can also act as enzymes.
Thousands of different enzymes catalyze the vast range of reactions that take place within cells, but each enzyme typically supports one of the following types of tasks:
Continue reading “Science Snippet: Examining Enzymes”Quiz: Do You Know Your Immune System?
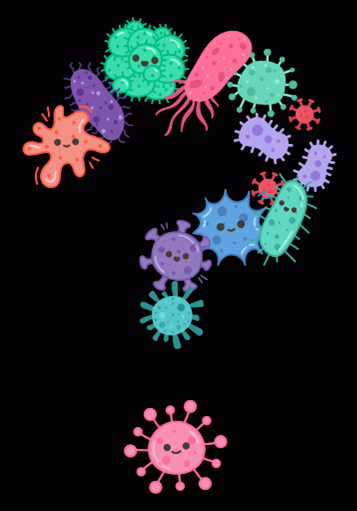
Throughout our immunology miniseries, we introduced the immune system and its many functions and components. Additionally, we highlighted how vaccines train your immune system, how the system can go awry, and how NIGMS-supported researchers are studying immunology and infectious diseases. Put your knowledge about the immune system to the test by taking the quiz below.
Continue reading “Quiz: Do You Know Your Immune System?”Research Organism Superheroes: Axolotls
The friendly-looking axolotl (Ambystoma mexicanum) doesn’t seem to have much in common with its namesake, Xolotl—the Aztec god of lightning, death, and fire. In fact, axolotls can regrow lost limbs and other body parts like organs and parts of their central nervous systems—which goes against the concept of death!
Continue reading “Research Organism Superheroes: Axolotls”