To get a look at cell components that are too small to see with a normal light microscope, scientists often use cryo-electron microscopy (cryo-EM). As the prefix cryo- means “cold” or “freezing,” cryo-EM involves rapidly freezing a cell, virus, molecular complex, or other structure to prevent water molecules from forming crystals. This preserves the sample in its natural state and keeps it still so that it can be imaged with an electron microscope, which uses beams of electrons instead of light. Some electrons are scattered by the sample, while others pass through it and through magnetic lenses to land on a detector and form an image.
Typically, samples contain many copies of the object a scientist wants to study, frozen in a range of orientations. Researchers take images of these various positions and combine them into a detailed 3D model of the structure. Electron microscopes allow us to see much smaller structures than light microscopes do because the wavelengths of electrons are much shorter than the wavelength of light. NIGMS-funded researchers are using cryo-EM to investigate a range of scientific questions.
Caught in Translation
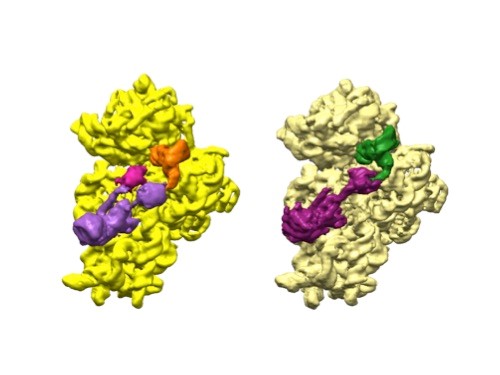
Joachim Frank, Ph.D., a professor of biochemistry and molecular biophysics and of biological sciences at Columbia University in New York, New York, along with two other researchers, won the 2017 Nobel Prize in Chemistry for developing cryo.
Dr. Frank’s lab focuses on the process of translation, where structures called ribosomes turn genetic instructions into proteins, which are needed for many chemical reactions that support life. Recently, Dr. Frank has adopted and further developed a technique called time-resolved cryo-EM. This method captures images of short-lived states in translation that disappear too quickly (after less than a second) for standard cryo-EM to capture. The ability to fully visualize translation could help researchers identify errors in the process that lead to disease and also to develop treatments.
Preventing Kidney Stones
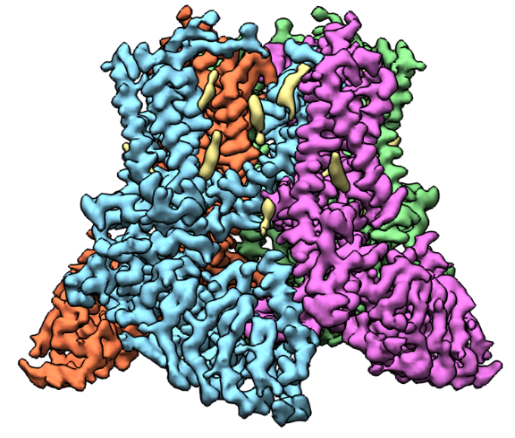
Vera Moiseenkova-Bell, Ph.D., an associate professor of pharmacology at the University of Pennsylvania Perelman School of Medicine in Philadelphia, researches transient receptor potential (TRP) channels using cryo-EM. TRP channels have been implicated in a diverse range of cellular processes, including pain and temperature sensation, neuronal development, heart and kidney disease, and cancer.
One focus of Dr. Moiseenkova-Bell’s lab is a channel, called TRPV5, primarily found in kidney cells that is essential for reabsorbing calcium into the blood. If it malfunctions, the amount of calcium in urine greatly increases, possibly leading to kidney stones. Dr. Moiseenkova-Bell’s research in this area has provided molecular details of how TRPV5 is modulated and identified a molecule that can inhibit the channel. Currently, her laboratory is using cryo-EM to determine TRPV5 channel structures in conditions that better mimic those found in the body, including the presence of regulatory proteins. She hopes that a better understanding of how this channel works will inform the search for drugs that effectively treat kidney stones.
Bacterial Wires and Medical Devices
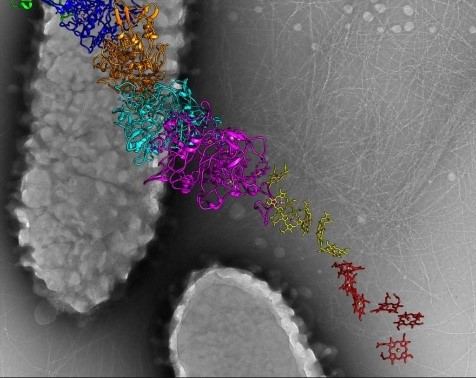
Edward Egelman, Ph.D., a professor of biochemistry and molecular genetics at the University of Virginia in Charlottesville, uses cryo-EM to study type 4 pili, appendages that help certain bacteria enter tissues.
In 2019, Dr. Egelman’s lab helped answer a question surrounding the Geobacter sulfurreducens bacterium, which conducts electricity. Some scientists had suggested that the electricity flowed through type 4 pili. But Dr. Egelman showed through cryo-EM that the electricity is conducted by nanowires, or chains of a unique protein that each surround a core of iron-containing molecules. Knowing the structure of G. sulfurreducens’ nanowires could enable researchers to design tiny wires, about 1/100,000th the width of a human hair, to connect miniature pacemakers and other implantable electronic devices directly to living tissues.
Understanding Cilia’s Rhythm
Daniela Nicastro, Ph.D., a professor of cell biology at the University of Texas Southwestern Medical Center in Dallas, conducts research using a form of cryo-EM called cryo-electron tomography (cryo-ET) to study relatively large cell structures—those that are too big for standard cryo-EM—at high resolution. Cryo-ET requires a couple of additional steps to prepare samples, which are then tilted as they are imaged in an electron microscope. This enables researchers to create a 3D model by combining the various tilt views of one object, whereas standard cryo-EM combines images from many copies of an object in different orientations.
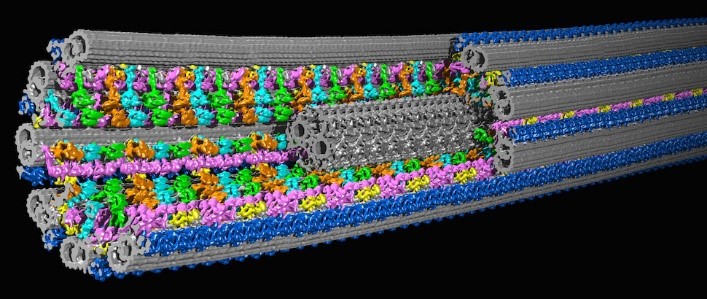
Dr. Nicastro studies organelles, including cilia. Cilia (cilium in the singular) are found in many living things, from single-celled algae to human cells, and their rhythmic beating can help propel cells or remove debris. Defects in human cilia can cause chronic respiratory disease, impaired fertility, brain development defects, congenital heart disease, and other illnesses.
Dr. Nicastro focuses on one of cilia’s many parts, a protein called dynein that drives its movement. By investigating dynein and associated proteins with cryo-ET, Dr. Nicastro hopes to shed light on how healthy cilia move and how their movement is disrupted in cilia-related diseases, which is an essential first step in developing treatments.
Ion Channels and Nausea Treatment
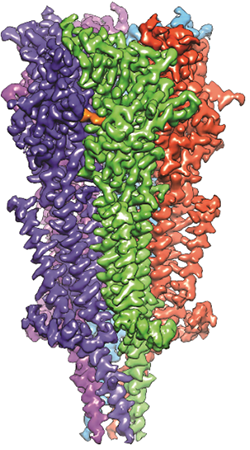
Sudha Chakrapani, Ph.D., a professor of physiology and biophysics at Case Western Reserve University School of Medicine in Cleveland, Ohio, uses cryo-EM to study how changes in ion channels’ structures affect the way they work. Ion channels are proteins in cell membranes that help regulate many processes such as motor function and pain transmission, and medications often target them.
Recently, Dr. Chakrapani’s lab identified a structure of an ion channel, known as the full-length serotonin receptor, that binds to a group of drugs that can manage nausea caused by cancer therapies. Understanding how these medications interact with the full-length serotonin receptor could help researchers further improve their designs. This insight would be valuable because while these antinausea drugs work for many patients, there’s still a significant portion that they don’t help.
NIGMS supports cryo-EM research through grants R01GM055440 (Frank), R35GM122510 (Egelman), R01GM129357 and R01GM103899 (Moiseenkova-Bell), R01GM083122 (Nicastro), and R01GM108921 and R35GM134896 (Chakrapani).