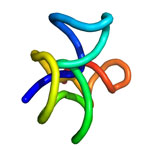
Roughly half the world’s population is now at risk for mosquito-borne diseases other than malaria, such as yellow fever, West Nile virus and dengue fever. These three diseases are caused by flaviviruses, a type of virus that carries its genetic material as a single strand of RNA.
Flaviviruses have found a way not only to thwart our bodies’ normal defenses, but also to harness a human enzyme—paradoxically, one normally used to destroy RNA—to enhance their disease-causing abilities. A team of scientists led by Jeffrey Kieft at the University of Colorado at Denver found that flaviviruses accomplish both feats by bending and twisting a small part of their RNA into a knot-like structure.
The scientists set out to learn more about this unusual ability. First, they determined the detailed, three-dimensional architecture of the convoluted flaviviral RNA. Then, they examined several different variations of the RNA. In doing so, they pinpointed parts that are critical for forming the knot-like shape. If researchers can find a way to prevent the RNA from completing its potentially dangerous twist, they’ll be a step closer to developing a treatment for flaviviral diseases, which affect more than 100 million people worldwide.
This work also was supported by the National Institute of Allergy and Infectious Diseases and the National Cancer Institute.
Learn more:
University of Colorado News Release
Kieft Lab