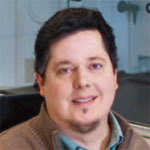
Fields: Neuroscience, assistive technology design
Works at: Purdue University, West Lafayette, IN
Hobbies: Gadgetry, architectural design
Bizarre collectible: Ecuadorian shrunken head (not a real one—it’s a replica made from goatskin)
Credit: Andrew Hancock, Purdue University
At the age of 18, Brad Duerstock had a devastating accident. A star member of his high school swim team, Duerstock hit his head during practice in a way that broke his neck and paralyzed all of his limbs. Today, he studies spinal cord injuries much like his own, investigating how the damage occurs and how it could possibly be repaired.
Duerstock has worked to make science accessible to people with disabilities, whether they use wheelchairs, as he does, or have visual or other impairments. For example, he has redesigned laboratory space to make it easier for people with disabilities to navigate and perform tasks.
“I like knowing that what I do can ultimately impact others,” Duerstock says.
Duerstock’s Findings
Much of Duerstock’s research deals with what occurs immediately following a nerve injury. In a spinal cord injury, nerve tissue becomes severed or dies. The immune response and bleeding in the injured area can cause extra damage to nerves in the spinal cord. Duerstock and his team have found that a molecule called acrolein is produced in spinal cord injuries and that it kills the nerves it encounters as it spreads around the injury site. They have been investigating a compound called polyethylene glycol (PEG), a polymer that could seal ruptured nerve cell membranes, possibly protecting nerve tissue from further damage immediately following a spinal cord injury.
Duerstock also founded and leads the Institute for Accessible Science (IAS), a community of scientists, students, parents and teachers whose goal is to promote better accommodations for people with disabilities who are studying or working in the sciences. The IAS looks into how to redesign lab spaces and equipment to increase accessibility for people with disabilities, particularly those with limited mobility or vision.
Although Duerstock originally wanted to be a doctor, he believes his true calling is in research. “The sense of discovery and the impact on others are big motivations for me,” says Duerstock. “Being a researcher, you might have a broader impact on society than you would as a practicing physician.”
Content adapted from the NIGMS Findings magazine article Opening Up the Lab.