This time of year, lights brighten our homes and add sparkle to our holidays. Year-round, scientists funded by the National Institutes of Health use light to illuminate important biological processes, from the inner workings of cells to the complex activity of the brain. Here’s a look at just a few of the ways new light-based tools have deepened our understanding of living systems and set the stage for future medical advances.
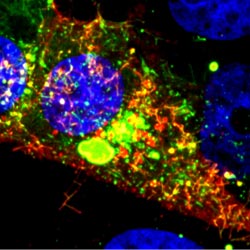
Visualizing Viral Activity
What looks like a colorful pattern produced as light enters a kaleidoscope is an image of a cell infected with respiratory syncytial virus (RSV) lit up by a new fluorescent probe called MTRIPS (multiply labeled tetravalent RNA imaging probes).
Although relatively harmless in most children, RSV can lead to bronchitis and pneumonia in others. Philip Santangelo of the Georgia Institute of Technology and Emory University, along with colleagues nationwide, used MTRIPS to gain a closer look at the life cycle of this virus.
Once introduced into RSV-infected cells, MTRIPS latched onto the genetic material of individual viral particles (in the image, red), making them glow. This enabled the researchers to follow the entry, assembly and replication of RSV inside the living cells.
The knowledge gained from the new imaging technique could help scientists develop antiviral drugs or vaccines that might limit the spread of RSV. Scientists may also be able to use the approach to study other viruses, such as flu and Ebola.
Lighting up Brain Cells
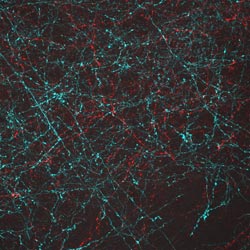
The nerve cells, or neurons, in this image of mouse brain tissue are lit up in blue and red by light-sensitive proteins called opsins that were originally discovered in algae.
To study how specific groups of neurons affect brain activity, scientists use opsins as switches for turning the cells on and off. First, they direct the neurons to produce particular opsins, most of which react to light in the blue-green range. They then shine light on the cells. The opsins sense the light and respond by altering the electrical voltage of the neurons. Depending on the opsin selected, the change in voltage will either turn the neuron off or activate it.
A research team led by Ed Boyden of the Massachusetts Institute of Technology and Gane Ka-Shu Wong of the University of Alberta discovered an opsin that responds primarily to red light. As shown in this image, the scientists can use the different opsins to manipulate two groups of neurons simultaneously, providing a more comprehensive look at how the two sets of brain cells interact.
An additional benefit of the new opsin is that the red light used to trigger its activity is less damaging to tissue than blue-green light. This trait may make the opsin a useful tool for scientists exploring ways to treat degenerated retinas; results from animal studies have already shown that some opsins, when inserted into retinal cells, can help restore light sensitivity.
Tracking a Key Player in Cancer Spread
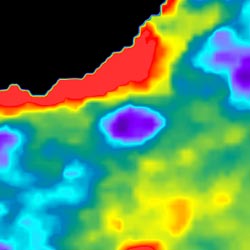
In this image of a cancer cell, researchers used a new biosensor to light up Rac1, a molecule involved in cell movement and the spread of cancer cells to other parts of the body.
Cancer cells travel from a primary tumor using invadopodia, foot-like protrusions that break through surrounding connective tissue. Invadopodia are driven by protein filaments that repeatedly grow and disassemble.
Researchers led by Louis Hodgson of Albert Einstein College of Medicine suspected that Rac1 might play a role in this construction-breakdown cycle, so they developed a fluorescent biosensor to track its activity. They then used the biosensor to study highly invasive breast cancer cells taken from rodents and humans. The scientists observed invadopodia forming when Rac1 activity was low and disappearing when it was high. They then confirmed their findings when they shut down the gene responsible for Rac1 and saw the invadopodia remain intact indefinitely.
Researchers now hope to find a way to turn off Rac1 in cancer cells without disrupting its function in the rest of the body.