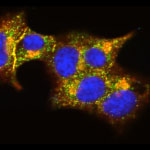
View larger image
The identities of the proteins that drive insulin production and release from pancreatic beta cells have largely been a mystery. In new work from the lab of William Balch of the Scripps Research Institute, researchers isolated and then identified all the insulin-bound proteins from mouse beta cells. The results provided a roadmap of the protein interactions that lead to insulin production, storage and secretion. The researchers used the roadmap to identify a protein called TMEM24, which was abundant in beta cells and binds readily to insulin. Balch and his team uncovered that TMEM24, whose involvement in insulin secretion was previously unknown, effectively regulates slower insulin release and could have a key role in maintaining control of glucose levels in the blood. The scientists hope that this roadmap of insulin-interacting proteins will lead to the development of new, targeted approaches to treating type 2 diabetes and a similar insulin-related condition called metabolic syndrome.
Learn more: