In part II of this series, we reveal how the ECM helps body cells move around, a process vital for wounds to heal and a fetus to grow. Here we introduce the extracellular matrix (ECM) and discuss how it makes our tissues stiff or squishy, solid or see-through.
When we think about how our bodies are made and what they do, we usually focus on organs, tissues and cells. These structures have well-known roles. But around, within and between them is a less understood material that also plays an essential part in making us what we are.
This gelatinous filler material is known as the extracellular matrix (ECM). Once thought to be the biological equivalent of bubble wrap, we now know that the ECM is a dynamic, physiologically active component of all our tissues. It guides cell shape, orientation and function.
The ECM is found in all of our body parts. In some tissues, it’s a thin layer separating cells, like mortar between bricks. In other tissues, it’s the major constituent.
The ECM is most prevalent in connective tissue, the material that forms our skeletons, cushions our internal organs and winds between blood vessels and around nerves. In connective tissue, the ECM is more abundant than the cells suspended within it.
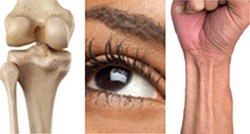
What makes the ECM truly unique is its variability: Its texture, composition and functions vary by body part. That’s because the ECM’s deceptively simple recipe of water, fibrous proteins and carbohydrates has virtually endless variations.
In general, the fibrous proteins give the ECM its texture and help cells adhere properly. Carbohydrates in the ECM absorb water and swell to form a gel that acts as an excellent shock absorber. Continue reading “The Extracellular Matrix, a Multitasking Marvel”