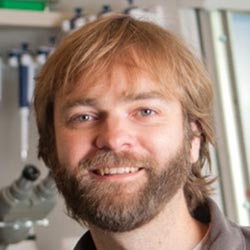
Credit: Kristan Jacobsen
Fields: Evolutionary genetics, virology, microbiology, cell biology
Works at: University of Utah, Salt Lake City
When not in the lab, he’s: Gardening, supervising pets, procuring firewood
Hobbies: Canoeing, skiing, participating in facial hair competitions
“I really look at my job as an adventure,” says Nels Elde. “The ability to follow your nose through different fields is what motivates me.”
Elde has used that approach to weave evolutionary genetics, bacteriology, virology, genomics and cell biology into his work. While a graduate student at the University of Chicago and postdoctoral researcher at the Fred Hutchinson Cancer Research Center in Seattle, he became interested in how interactions between pathogens (like viruses and bacteria) and their hosts (like humans) drive the evolution of both parties. He now works in Salt Lake City, where, as an avid outdoorsman, he draws inspiration from the wild landscape.
Outside the lab, Elde keeps diverse interests and colorful company. His best friend wrote a song about his choice of career as a cell biologist. (You can hear this song at the end of the 5-minute video in which Elde explains his work.)
Elde’s Findings
The key research question for Elde’s team is: “How have pathogens shaped the composition of our genomes and the complexity of pathways in our cells?” And, on the other hand: “How do viruses adapt to overcome host defenses?”
Many viruses are tiny—much smaller than bacteria. They are essentially only genetic material (DNA or RNA, depending on the type of virus) wrapped inside a protein shell. Unlike bacteria, which are self-contained living organisms, viruses can only reproduce by hijacking the cellular equipment of a living organism host.
Elde and his research group focused on Vaccinia, the DNA virus best known as the smallpox vaccine. They discovered that this virus has an interesting characteristic, which they call a “genomic accordion.” When under acute threat, Vaccinia’s genome gets bigger. When danger subsides, its genome returns to its normal size.
This accordion-like expansion and contraction of Vaccinia’s genome begins randomly—as the virus replicates, it can gain extra copies of genetic segments, including entire genes. Some of these genes might help it survive the immediate threat.
Elde and his research team have observed that Vaccinia’s genomic accordion allows it to take advantage of natural selection. Enlarged areas of the genome provide a bigger target for genetic changes (point mutations). If one of these mutations helps the virus persist, Vaccinia is likely to retain the change even after shrinking its genome back to size.
Elde’s group proposes that these strategies allow Vaccinia to withstand host immune defenses and maybe even other environment stressors, like toxic chemicals or changes in temperature, UV light or moisture. The scientists expect that all pox viruses—and possibly other viruses with double-stranded DNA—have genomic accordions.
On the other hand, the situation is different for RNA viruses, such as those that cause HIV, Ebola, hepatitis C, measles and influenza. RNA viruses have more streamlined genomes. Instead of structural genomic changes, they adapt to environmental challenges by multiplying rapidly, generating many random point mutations, and relying on the odds that some of these mutations will be beneficial.
Elde’s team saw Vaccinia’s genomic accordion in action when they put the virus in a dish containing laboratory-grown human cells. Upon infection, the human cells produce Protein Kinase R, a protein that prevents viruses from replicating.
In response, Vaccinia accumulated extra copies of a gene, called K3L, that counteracts the human protein, bolstering replication. The scientists found that after a relatively short time (less than 100 viral generations), some Vaccinia virus particles had already accumulated 15 copies of this antidote gene, effectively increasing the size of their genomes by up to 10 percent. Some virus particles had also incorporated a point mutation that provided an additional advantage.
The concept of an expanding viral genome contradicts the common thinking that viruses do better with the smallest possible genomes.
“We often think of viruses as these streamlined entities with genes that are occupying high-value genomic real estate, so to speak,” Elde says. “But instead of getting smaller, it seems that for DNA viruses, the genome is getting larger—at least temporarily.”
As Elde’s team continues to investigate these phenomena, he finds the feeling of adventure only becoming greater. “The stuff we’re doing today we had no idea we’d be doing 5 years ago,” he says. “Being able to move rapidly between microbial and evolutionary genetics is invigorating—it’s a fun and messy process.”